From Gasoline to Hyacinth
Maciej Szaleniec
Jerzy Haber Institute of Catalysis and Surface Chemistry Polish Academy of Scienecs, Niezapominajek 8, 30-239 Kraków
For more than two centuries, scientists and technologists have been seeking better and cheaper methods of chemical synthesis using catalysts – molecules that can accelerate and boost the efficiency of certain chemical processes. But over millions of years of evolution, Nature itself has developed extremely specialized catalysts, called enzymes. Chemists are nowadays looking more and more to such extant bio-catalysts found in living organisms.
Chemical synthesis constitutes the largest sector of industry in Europe. According to the CEFIC 2006 report, as much as 80% of all industrial processes nowadays involve catalysts, which assist in the manufacture of better and cheaper fuels, various types of plastic, cleaning products and medications. Catalysts are also used in environmental protection, e.g. reducing fume emissions in every car.
How do catalysts work?
How might a catalyst lower production costs? Firstly, it may speed up a chemical reaction. In the manufacturing process, we would like to be able to produce large quantities of a given compound relatively quickly. One way of accelerating such a reaction is to carry it out at a higher temperature. However, when a catalyst is applied, it is possible to lower the reaction temperature, thereby saving energy. Secondly, a catalyst can cause a certain “reaction path” to become more privileged. This means that only a single, desired compound is produced, avoiding the synthesis of chemical wastes. A catalyzed production process can be significantly cheaper, as well as significantly more ecological in view of the energy savings and minimal waste production.
An ideal catalyst should operate at room temperature, in a non-toxic environment, and stimulate the production of only a single, desired product. However, such a catalyst is very difficult to obtain by chemical methods. Fortunately, Nature has done quite a bit of that work for us – having created enzymes, which meet all these conditions and catalyze millions of chemical reactions that occur inside living organisms.
These biocatalysts are extraordinary complex biological polymers. Most of them are protein enzymes (consisting of combined amino acids), although there are also known biocatalysts which have nucleic acids as their main component (such as ribozymes). The biopolymer thread of an enzyme, folding into a complex pattern, forms a stable skeleton which encircles an active centre, where the catalytic reaction occurs. Enzymes are very productive and selective catalysts – they operate at room temperature, in a water environment, and usually synthesize only a single product. We are unfortunately still unable to synthesize enzymes out of individual amino acids.
Instead, we use microorganisms to do the whole job for us. First, a DNA segment encoding the synthesis of a given enzyme is first implanted into bacteria. Then, after the bacteria colony is grown, its biosynthesis is stimulated. This causes the bacteria to produce a complete enzyme, which can then be purified and used for carrying out the desired reaction. While there is significant progress in this field, we are still not able to catch up with Nature. Therefore, when it comes to obtaining proteins, we rely on their natural sources. Also for ethical reasons, we try to avoid the isolation of enzymes from higher animals. Instead, we use microorganisms to do the whole job for us. For this purpose, both natural (that is, genetically unmodified, such as baker’s yeast), as well as genetically modified organisms, are used. In order to use bacteria for the production of the desired enzyme, To achieve this, a DNA segment encoding the synthesis of a given enzyme is first implanted into bacteria. As a result, the bacteria produce a finished enzyme, which can then be purified and used for the selected reaction, or even whole bacteria (like small capsules filled with a catalyst) can be used in the process. It is these recombinant enzymes that can be found not only in industrial reactors but also in our washing liquids (where proteases and lipases remove protein and fat stains) or in kitchens (for the production of homemade cheese or meat softening).
From the Weser bottom to the lab
At the Jerzy Haber Institute of Catalysis and Surface Chemistry, Polish Academy of Sciences, we study a fairly atypical enzyme called ethylbenzene dehydrogenase. It comes from the bacterium Aromatoleum aromaticum (EbN1 Azoarcus sp.), discovered in the bottom sediments of the Weser River near Bremen in Germany. This bacterium lives in an anaerobic environment, feeding upon substances that are poisonous to most living organisms: toluene, ethylbenzene, and propylbenzene. Ethylbenzene dehydrogenase catalyzes the reaction whereby ethylbenzene is oxidized into (S)-1-phenylethanol. Why is this reaction unusual? Firstly, under anaerobic conditions, the enzyme is unable to utilize molecular oxygen in the oxidizing reaction, as happens in most organisms capable of oxidizing hydrocarbons. Instead, it uses a special molybdenum cofactor present in its active centre, which obtains oxygen from water. After two protons detach from the water bound to the molybdenum atom what is left is an oxygen ligand (so-called oxo group) which is able to oxidize the substrate. Secondly, the industrially important feature of the reaction is that only a single optical isomer with an S configuration is produced.
Optical isomers (Fig. 1) are compounds which have identical physiochemical properties, aside from the fact that they alter the polarization of light passing through them in opposite directions. A small difference? Well, as far as biological action is concerned, such optical isomerism is in fact extremely important. All the amino acids which comprise living organisms are built solely from one type of optical isomer (called “enantiomers”), and no terrestrial organisms are able to utilize amino acids of the other configuration in biosynthesis. Our senses of smell and taste can also distinguish between enantiomers very well, e.g. (S)-1-phenylethanol, used in the food industry as a scent additive, smells delicately of hyacinth with a detectable note of gardenia and strawberries, while its mirror image, called (R)-1-phenylethanol, exudes a honey-floral smell and is reminiscent of unripe fruit in the taste.
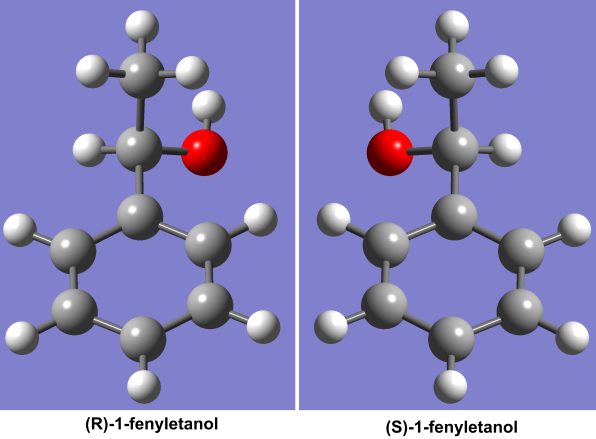
Fig. 1. Enantiomers – molecules which are identical except being mirror images of one another – can sometimes demonstrate surprisingly different properties.
Different optical isomers can have different effects not only on our senses but also on our health. Frequently, one optical isomer has therapeutic properties while the other may cause undesirable side effects. That is why the pharmaceutical industry is interested in compounds with high optical purity, which can be used in the synthesis of new drugs.
We have discovered ethylbenzene dehydrogenase to be a highly versatile enzyme, able to catalyze the oxidation reaction of as many as 30 different compounds structurally similar to ethylbenzene. In each case, alcohol is produced – most likely in a way analogous to the synthesis of 1-phenylethanol.
Thanks to this discovery, Nature has given us a tool able to produce 30 new alcohols [1] which could prove useful not only as scents for perfumes and creams but above all as building blocks for new drugs design. We expect the enzyme to demonstrate a high selectivity for only a single optical isomer, both in ethylbenzene oxidation and for the other substrates. How does ethylbenzene dehydrogenase perform these extraordinary reactions? In order to solve that intriguing riddle, we are also studying the possible reaction mechanisms by theoretical methods. Thanks to complex calculations that required the use of supercomputers from the CYFRONET Academic Computer Center, it was possible to discover how the oxo ligand detaches the hydrogen atom from the hydrocarbon (Fig. 2) and then the OH group is separated from the molybdenum centre and attached to the substrate, forming alcohol. The enzyme has a specially carved active centre in a shape that allows the substrate to position only one way towards the molybdenum centre [2]. It is thanks to this that the enzyme is highly selective, as oxidation only takes place on one side of the molecule. Such knowledge turned out to be much more valuable than the ability to transform the smell of a gas station into a delicate scent of hyacinths because ethylbenzene dehydrogenase turned out to be the first enzyme from a whole group of very interesting molybdenum catalysts. One of them may soon be producing drugs that are valuable to us.
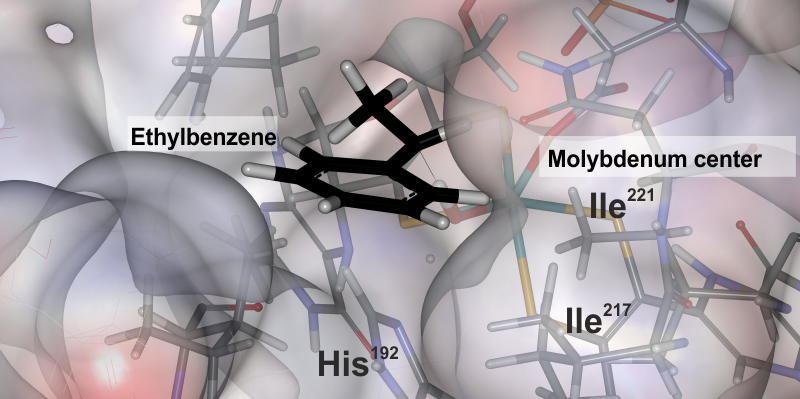
Fig. 2. Oxo ligand of molybdenum cofactor activates ethylbenzene only from one side [2]
Literature
[1] M. Szaleniec, C. Hagel, M. Menke, P. Nowak, M. Witko, J. Heider, “Kinetics and mechanism of oxygen-independent hydrocarbon-hydroxylation by ethylbenzene dehydrogenase”, Biochemistry 2007; 46, 7637-7646
[2] M. Szaleniec, A. Dudzik, B. Kozik, T. Borowski, J. Heider, M. Witko, “Mechanistic basis for the Enantioselectivity of the Anaerobic Hydroxylation of Alkylaromatic compounds by Ethylbenzene Dehydrogenase”, J. Inorg. Biochem., 139 (2014) 9-20